David M. Delaney
Ottawa, Canada
June 10, 2006
Key words: passive survivability, solar
air heater, thermosyphon, passive solar, heat store, thermal closet,
thermal storage wall, heat store
wall, thermal mass, air-to-air heat exchanger, solar heat, solar
thermal, natural convection, forced convection, plastic film flapper, plastic film damper,
dampers, rock bed, bed of stones, packed bed, gabion, column of
stones, attic, basement, high temperature heat store, segregated heat
store, passive collection, passive charging, passive
discharging, indoor air quality, IAQ, mould, mold, earthquake.
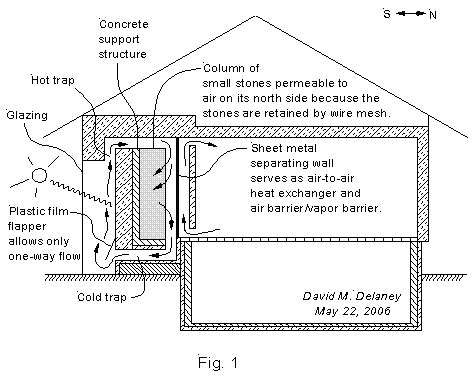
The
entirely passive residential solar heating system described here was
conceived for passive survivability, with particular attention to
indoor
air quality and earthquake hazard. The proposed heating system could
provide all of the
space
heat needed by a well insulated house in a cold winter, even during a
prolonged absence of electricity and other non-solar energy. The
system,
Fig. 1, consists of a solar air heater on the south wall,
a high
temperature heat store built into the south wall, and a passive
air-to-air
heat exchanger that separates the air of the heating system from the
air of the living space.
Heat is
transferred from the heat store to the heat exchange
wall by radiation from the thermal mass of the heat store and
by
contact between the air of the heat store and the heat exchange
wall. The heat exchange wall has a large surface area equal to
the
area of the north face of the thermal mass. The surfaces of the heat
exchange wall have a high emissivity at the wavelength of the
temperature of the thermal mass to facilitate reception of radiative
heat transfer from the thermal mass and re-radiation north to the south
wall of the insulated wall of the living space.
Heat is transferred from the heat exchange wall to the living space air
by
contact between the sheat exchange wall and the air of the
living space in the heat exchanger, and by contact between the air of
the living space inside the heat exchanger and the south surface of the
insulated wall that divides the living space from the heat exchanger.
This south surface of the insulated wall is heated by radiation from
the heat exchange wall. The
two surfaces of the heat exchange wall and the south surface of
the
insulated wall separating the heat exchanger from the living space
should be painted
with paint known to have very high emissivity (emissivity = 0.9) at
thermal wavelengths. For the same reason, the north surfaces of the
stone columns and their steel retaining mesh may be sprayed
with the same paint to raise their emissivity to about 0.9.
The heat exchange wall might be corrugated or folded to increase its surface area.
See the MathCAD file (rendered to PDF form) in which equations are solved and graphs produced
here (PDF).