(last updated: Feb 01, 2009)
The polar ice caps contain enough water to raise sea level by seventy
meters above today's sea level. This page describes the various polar ice caps, investigates
the melting of the much larger ice caps that covered the northern
hemisphere during the last ice age, and attempts to estimate how
quickly the ice caps will melt in response to rising temperatures from
global warming.
The chart below (from Science
Aug 2006) shows the reservoirs of water on Earth, and the
fluxes
between them. The unit are in thousands of cubic kilometers
(103
km3). Note
the large quantity of water locked up in glaciers and snow compared to
the other (non-ocean) water reservoirs.

Global hydrological fluxes (1000 km3/year)
and storages (1000 km3) with natural and
anthropogenic cycles are synthesized from various sources (1,
3–5).
Big vertical arrows show total annual precipitation and
evapotranspiration over land and ocean (1000 km3/year),
which include annual precipitation and evapotranspiration in major
landscapes (1000 km3/year) presented by small
vertical arrows; parentheses indicate area (million km2).
The direct groundwater discharge, which is estimated to be about 10% of
total river discharge globally (6),
is included in river discharge.
The oceans cover 71% of the Earth's surface (3.5 x 1014
m2), with an average
depth of 4
km. This amounts to 1.4 x 1024
g, or 1.4 x 109
km3 of water.
Most
water on Earth (97.25%)
is stored in the oceans, 2.05% in ice (90% of which resides in the
Antarctic ice sheet, 9% or 2.5 x 106
km3 in Greenland), with the remainder stored as
ground water
(0.68%), while the amount of water in lakes and rivers, as soil
moisture, in the biosphere and atmosphere is insignificant.
The total volume of water in the atmosphere is about 1.3 x 104
km3 [ref]
This chart may be missing most of the Earth's water. Estimates of
how much water is stored in the mantle vary from one third of the ocean
to one or two oceans [ref].
Water enters the mantle via the subduction due to plate tectonics, and
returns to the surface by volcanic emissions. Of course, this happens
over a very long time frame, so it has little interaction with the
water cycle described above.

There are two sources of sea level change. Eustatic change is
caused by water entering or leaving the ocean (usually from ice caps),
or large scale changes to the sampe of the ocean floor.
Isostatic
change is caused by the local rising and falling of the coastline
because of geoligical forces, and
is not an indicator of global sea level.
There are three main causes of Eustatic sea level rise:
- Thermal Expansion - Water expands slightly as it gets
warmer.
This is presently the main cause of rising sea levels.
A 3
°C rise of temperature will increase the thickness of a 100
m layer of sea water in low latitudes by around 9 cm.[ref],
or a 1
°C rise in the top 1 km of ocean will cause 20 cm sea level rise.
- Melting Mountain Glaciers - Mountain glaciers in temperate
regions are shrinking as climate warms. Their total volume is
relatively small, so they are contributing a smaller portion of sea
level rise. The total amount of glacier ice left is actually only
enough to raise sea level by 15-37 cm. [ref]
- Melting Polar Ice Sheets - The polar ice sheets are melting
around the edges, but growing in their centers due to increased
snowfall. These two process were roughly in balance
in the 20th century, but that is not longer the case.
Averaged globally and throughout the entire water column, the
temperature of the ocean has only risen by 0.04ºC since 1955.
So
far only the surface mixed layer with a thickness of a few hundred
metres has warmed, while the average ocean depth is 3800m. [ref]
Sea level is believed to be rising worldwide by 1.7 millimeters per
year over the last century, and 2.8 ± 0.4 mm/year
this decade [Science,
Aug 11, 2006 p 827]. This is due to the expansion of warming
water and the added outwash from
melting glaciers in Greenland, Alaska, tropical highlands and elsewhere
in Antarctica.
The IPCC estimates that ocean levels rose by 10 to 20 cm in the 20th
century. However, other factors such as wind patterns and
ocean
currents affect local sea level, so this rise is not uniform.
For
example, an El Nino can temporarily change ocean levels by up to 60 cm.
in some regions. The
map below shows sea level changes, measured by satellite since 1980 and
calculated before that. Some parts of the ocean are
experiencing
falling sea levels in contrast to the general trend. Note the
black triangles indicate tidal
guages - most of them are located in areas with the fastest
rising sea level.
This has led to overestimates of sea level rise in the past.

An ice cap consists of a large mass of ice, which exerts a
gravitational attraction on the sea water that surrounds it, causing
the local sea level to rise. When some of the ice melts, the meltwater
is distributed around the entire ocean, but the reduction in
gravitational attraction is felt locally. Local sea level will actually
fall as the ice cap melts. See [Science
Mar 2002]. The map below shows the relative sea level change
from the melting of the southern one-third of the
Laurentide Ice Sheet that covered Canada during the last ice age. The
dark blue is the region of sea level fall.

Numerical Measures Used for
Ice Cap Melting and Sea Level Rise |
- One Gigatonne (Gt), or one billion tons of water =
one cubic kilometer (1 km3)
- One meter of sea level rise inundates about 1011
square meters of land, about
0.07% of the land area of the earth.
- Water flow is measured in Sverdrups, where 1 Sv = one
million cubic meters per second.
The Amazon River flows at 0.3 Sv, the Gulf Stream at 20 Sv.
|
Gigatonne,
or cubic kilometer (km3) |
Millimeter
(mm) of Sea Level |
Sverdrups
(Sv) |
1 Gt / year |
0.0028 mm / year |
3.22 x 10-6
Sv |
361 Gt / year |
1 mm / year |
0.011 Sv |
31,000 Gt / year |
85.9 mm / year |
1 Sv |
There are three major ice sheets on Earth, which could raise sea level
by 78 meters in the unlikely event that they all melted (as shown here). Annual
snowfall on the ice sheets is equivalent to
6.5 mm of sea level [ref].
The
present sea level is 120 m above that at the end of the last ice age,
when the global average temperature was about 6° C colder than
today, and large parts of the Earth were covered by massive ice
sheets. Global sea level stood about 6 m higher during the
last interglacial
period about 120,000 years ago, but this event cannot be directly
linked to any
ice shelf collapse. The temperature of the earth was perhaps
4-5° C warmer than
today 40
million years ago when the Antarctic ice sheet first made an
appearance. Sea level at that time was about 70 meters
higher than today.
Antarctica should be treated as three separate and distinct
regions: the Antarctic Peninsula, East Antarctic and West Antarctica.
Geologically these are three continental fragments that have
collided together (see this
PowerPoint slideshow), and they also have very different
climates. Ice
sheets on the Antarctic Peninsula are relatively small (enough ice to
raise sea level by a third of a meter [ref]),
and the climate there
is warming rapidly (6° C since 1950). It is misleading to apply
graphic examples of
warming there
to the rest of Antarctica. See this ice-free
image.
East Antarctica (65
meters
sea level equivalent) is by far the largest (up to 4 km thick) and most
stable of the ice caps. It began forming over 40 million
years
ago and has been stable for at least 14 million years, when the global
climate was significantly warmer than today. It would take a
local temperature rise of 17°
C to cause significant de-glaciation.
West Antarctica
(6 meters sea
level equivalent) is distinguished by the fact that most of it is
resting on bedrock below sea level. It is separated from the East
Antarctic ice sheet by a mountaing range. A continuous West Antarctic
ice sheet first appeared approximately 9 million years ago [ref].
It has suffered at
least one
complete collapse in the past, and has had multiple partial collapses
during the present interglacial period. Even though it is close the the
South Pole, this may be the ice sheet at most risk of collapse,
although no major change is expected for at least 100 years.
Over
the last decade it has added between 0.13 and 0.16 mm/year to global
sea level.
 |
|
The ice sheet covering West Antarctica is the last
great marine ice
sheet. Its bed lies below sea level and slopes down inland from the
coast. The profile shown is based on Thwaites Glacier, West Antarctica (11).
When the ice sheet is in equilibrium; influx from snowfall (q)
is balanced by outflow. A small retreat will provoke
changes in both the influx and the outflow. If these changes act to
promote further retreat, the ice margin is unstable.
But [Science
Mar 2007]
reports that sedimentation at the grounding line acts to stabilize the
ice sheet. At the current rate of sea-level rise, it would take several
thousand years to float the ice sheet off the bed. |
Greenland
(6.6 meters sea level
equivalent) is the furthest ice sheet from the pole, extending below
the Arctic Circle. The ice cap is presently melting around
the
edges (the elevation-change rate is –2.0
± 0.9 cm/year [ref])
and (maybe) growing in the center (6.4 ± 0.2 cm/year)
due to increased snowfall caused by warming temperatures. Most of
Greenland is above sea
level,
and it is almost surrounded by mountains (see this ice free image),
isolating the center from the
melting margins.
 |
 |

change in
cm / year
|
|
Antarctica |
Greenland |
|
|
|
|
|
 |
|
The
Ice Sheets at the Last Glacial Maximum 18,000 Years Ago
During
the last glacial maximum the ice sheets covered an area of 25 million
square kilometers, and were up to four kilometers thick. Sea
level
was about 120 meters lower than today. When they melted, and area of
about 20 million km 3
were submerged, about 10% of the global land mass, or an area larger
than South America. See this
detailed map and description.
|
|
Ice
Sheet |
Volume |
Potential
Sea Level Rise |
Present
Rate of Loss |
Sea
Level Equivalent |
References |
East Antarctica |
23.0 x 106 km3 |
67.3 meters |
0 ± 56 km3 /
year
-25 Gt / year (gain)
|
- 0.07 mm/yr
|
Science
Mar 2007 |
West Antarctica |
3.8 x 106 km3 |
5.0 meters (partly below sea level) |
148 ± 21 km3
/ year
50 Gt / year |
0.4 ± 0.2 mm/yr
0.14 mm/yr |
[ref]
Science
Mar 2007 |
Greenland |
2.46 x 106 km3 |
6.6 meters |
224 ± 41 km3
/ year
239 ± 23 km3
/ year
101 ± 16 km3
/ year |
0.62 mm/yr (my calculation)
0.54 mm/yr (their figure)
0.28 ± 0.04 mm / year |
Science
2005
Science
Sep 2006
Science
Nov 2006 |
Other glaciers |
0.68 x 106 km3 |
1.9 meters |
90 km3
/ year |
0.25 ± 0.11 mm/yr |
[ref] |
Although the world's glaciers only contain a fraction of the mass of
ice compared to the major ice sheets, they serve as a more sensitive
measure for local temperature changes. Detailed information on the
changing state of glaciers can be found at the World Glacier Mmonitoring Service.
Sea level is determined by the size of the polar ice caps. As
the
climate cools, water from the ocean is deposited as snow, and ice caps
grow as ocean levels drop, and as climate warms the ice melts and the
oceans rise. At any given temperature there is an equilibrium
level of ice cap size and sea level.
The table below identifies sea levels (compared to today) for several
time periods with different global average temperatures. It
can
be seen that there is roughly 6 meters of sea level rise for each
degree C of temperature rise. In a time of rapid temperature
change the ice does not melt all at once; it takes many hundreds or
possible several
thousands of years to reach equilibrium.
Date |
Period
Name |
Relative
Temperature |
CO2
( in ppm) |
Sea
Level |
Rise
mm / year |
Sea
Level
m / °C |
Comments |
next 1000 years |
Anthropocene |
+2 to +5° C |
500-1000 |
3.5 m ? |
3.5 |
|
Maximum rate from IPCC 2001 |
1993–2003 |
Late Holocene |
0° C |
380 ppm |
0 m |
2.6 ± 0.04 |
0 |
Measured by satellite. |
past fifty years |
|
-0.5° C |
|
|
1.8 |
|
Overpeck
et. al. |
13,800 - 7000
years |
|
|
|
11 |
|
The end of the last Ice Age |
14.2 - 13.7 Ky |
Meltwater
Pulse 1a |
|
|
|
40 |
|
Science: Mar
2002, Mar
2003 |
21,000 |
Last Glacial Maximum |
-4 to 7° C |
185 ppm |
–130 ± 10 m |
|
16 to 30 |
Science,
Jan 2007 |
130,000 |
Eemian Ingerglacial (MIS-5e) |
1 or 2° C |
290 ppm |
4-6 m |
20 |
2 to 6 |
See the discussion
below. |
425,000 to 375,000 |
Four Ice Ages Ago (MIS-11) |
about the same |
300 ppm |
up to 20 m |
slow |
|
Science,
July 2008, Wiki
(a) |
3 million |
Middle Pliocene |
2 or
3° C |
400-500 ppm |
25 ± 10 m |
|
5 to 10 |
USGS
Summary |
40 million |
Eocene, before Antarctic glaciation |
+5° C |
|
60 m |
|
12 |
|
100 million |
Cretaceous |
+8° C |
1000-2000 |
+ 80 m |
|
10 |
|
(a)
MIS-11 was a
stable interglacial period similar to the Holocene today, except that
it lasted for 50 thousand years. This extra time was all that was
required to melt most of the Greenland ice cap.
 | Sea Level Compared to Global Mean Temperature
This
chart shows four measurements of sea level at representative points of
time in the past. The points are roughly in a straight line, suggesting
a ratio of 100 m / 10° C, or about 10 meters of sea level rise for
every degree Celsius of temperature increase. This chart give no
indication of the amount of time it would take for a degree of warming
to increase sea level by that amount.
The red line is an estimate of the rate of sea level rise over a shorter time scale, as discussed here. |
The graph below shows that the melting rate of the world's ice caps
slows down as the climate becomes warmer (and CO2 levels become
higher). This is because the ice caps become smaller. The
lower chart shows the rapid melting of the glaciers in
the ten thousand years following the peak of the last ice age.
At certain times sea level was rising about half a meter per
year.
 |
Relation between estimated atmospheric CO2
and the ice
contribution to eustatic sea level indicated by geological archives and
referenced to modern (pre-Industrial Era) conditions.
(A) The
most recent time when no permanent ice existed on the planet
(sea level
= +73 m) occurred >35 million years ago when
atmospheric CO2
was 1250 ± 250 ppmV (54).
(B) In the early Oligocene ( 32 million years ago), atmospheric CO2
decreased to 500 ± 150 ppmV (54),
which was accompanied by the first growth of permanent ice on the
Antarctic continent, with an attendant eustatic sea-level lowering 45
±
5 m (55).
(C) Today. CO2
=
280 parts per million by volume (ppmV), eustatic sea level = 0
m.
Due to global warming, we are now slowly heading toward (B).
(D) The most
recent time of low atmospheric CO2
(185
ppmV) (56)
corresponds to the Last Glacial Maximum 21,000 years ago, when eustatic
sea level was –130 ± 10 m. |
 |
Time series of key variables encompassing the last
interval of
significant global warming during the last deglaciation, starting
20,000 years ago.
(A) Atmospheric CO2 from
Antarctic ice cores.
(B) Sea surface temperature in the western
equatorial Pacific based on Mg/Ca measured in planktonic foraminifera. [Is this the best proxy for
the temperature actually acting on the ice sheets?]
(C) Relative sea level as derived from several sites
far removed from the influence of former ice-sheet loading.
Note the two intervals with rapid melting, known as
a meltwater pulse (MWP). Deglacial sea-level rise
averaged 10 mm/year, but with variations including
two extraordinary episodes at 19,000 years before present
and 14.5 kyr B.P. when peak rates potentially
exceeded 50 mm/year (7–9).
Each of these "meltwater pulses" added the
equivalent of 1.5 to 3 Greenland Ice Sheets to
the oceans over a period of one to five centuries. |
The table below shows sea level rise for the 20th century, and
projections for the 21st century. The data suggest a sea level rising
rate of 3.4 millimeters/year per °C of global average
temperature increase. Observations from warmer times in the past show
that in the long term there is 5 to 10 meters of sea level rise for
each increase in global average temperature of one degree Celsius.
Dividing this by the present melting rate gives a melting time of 3,000
to 9,000 years, assuming a constant melting rate. However, it
is possible that melting rates will increase, so the ice cap melting
time may be shorter than that.
Perhaps we can get a better idea from the previous large global warming
event - the end of the ice age 20,000 years ago, which is shown in the
table above. A warming of 5 degrees over 10,000 years caused
an
essentially linear sea level rise over the same time period. [But why is there no sign of
the Younger
Dryas cooling
12,900 – 11,500 years before present, which occured in the
northern hemisphere where the major ice sheets were. The
equatorial Pacific warming looks nothing like the Greenland Ice Core record.]
 |
Rate of
Sea Level Rise in the 20th Century
Rate of sea-level rise obtained from tide gauge observations (red line)
and computed from global mean temperature (dark blue
line). The light blue band indicates the statistical error (one SD) of
the simple linear prediction (15).
|
Sea Level
in the 20th Century
Sea level relative to 1990 obtained from observations (red line,
smoothed) and computed from global mean temperature (blue
line). The red squares mark the unsmoothed, annual sea-level data. |
 |
Projections
of Sea Level Rise for the 21st Century
Past sea level and sea-level projections from 1990 to 2100 based on
global mean temperature projections of the IPCC TAR. The gray
uncertainty range spans the range of temperature rise of 1.4°
to 5.8°
C, having been combined with the best statistical fit. The dashed gray
lines show the added uncertainty due to the statistical error of the
fit.
Colored dashed lines are the individual scenarios as shown in (1);
the light blue line is the A1FI scenario, and the yellow line is the B1
scenario. |
In the last interglacial period, about 120,000 years ago, sea level was
probably 5 or 6 meters higher than today. There are suggestions that it
was still higher during the interglacial 400,000 years ago (Hearty
et
al., Geology, 27, 375, 1999). But to find a planet 2 or
3°C warmer than
now, as it will be this century in
“business-as-usual” scenarios, we
must go back to the middle Pliocene, about 3 million years ago. At that
time sea level was 25 ± 10 m greater than today. [ref]
On the other hand, conditions during the Eemian were very different
than today. As seen from this chart
of temperature anomolies
caused by the Milankovitch cycles, during the Eemian solar forcing in
the polar regions during the critical spring period was more than 60
watts per meter above normal. Compare this with 3.7 W/m2
of direct forcing from the doubling of carbon dioxide levels expected
at the end of the 21st century. It is quite possible that
this
was the cause of the extensive melting in the polar regions, and the
one or
two degrees of global temperature rise was mainly a side effect.
There is also a problem of using the Pliocene to predict the effects of
a similar warming today: The Pliocene was a time of cooling and ice cap
formation, while the near future is a time of warming and ice cap
melting. An ice cap creates its own regional climate by reducing local
temperature. For example, if you removed all of the ice from Greenland,
a new ice cap would not form at today's temperatures. This implies that
three degrees of warming will leave a much larger ice cap than the
Pliocene started with, so sea level increases may not be nearly so
high.
James Hansen thinks ice cap melting rates are a lot higher:
Some ice sheet
modelers believe that it requires millennia for ice sheets to respond
to forcing. I’m a modeler too, but I rate data higher than
models. Numerous recent studies show that when ice sheets
begin to
disintegrate sea level commonly rises at rates of a few meters per
century. [These
are
not the ice sheets that exist today.] Perhaps
even more
important, several independent studies with
independent methods, show that sea level does not change gradually with
the Earth’s orbital elements. Rather it exhibits rapid
changes of 10
meters or more on sub-orbital time scales. I refer, for example, to
studies of Siddall
et al. [also ref],
Potter et al., Thompson and Goldstein, to
name a few. Ice sheet models that move lethargically on millennial time
scales do not produce these real-world changes, because they are
missing critical physics. [ref]

Future evolution of the Greenland Ice Sheet calculated
from a 3D
ice-sheet model forced by three greenhouse gas stabilization scenarios.
The warming scenarios correspond to the average of seven IPCC models in
which the atmospheric carbon dioxide concentration stabilizes at levels
between 550 and 1000 ppm after a few centuries (4)
and is kept constant after that. For a sustained average summer warming
of 7.3°C (1000 ppm), the Greenland Ice Sheet is shown to
disappear
within 3000 years, raising sea level by about 7.5 m. For lower carbon
dioxide concentrations, melting proceeds at a slower rate, but even in
a world with twice as much CO2 (550 ppm or a
3.7° C summer
warming) the ice sheet will eventually melt away apart from some
residual glaciation over the eastern mountains.
Other Notes:
- The mid-Holocene may be a more comparable period to today,
which
experienced some warming, and evidence of sea level rises of a few
meters.
- If warming stabilises at
3 °C, the Greenland
ice sheet
could survive
for several thousand years. But if temperatures rise by
8 °C,
which several scenarios predict, then it would disappear in
1000 years. [ref]
- There has been no significant change in ice cap size in
Antarctica (east or west) since the 1950's [Science,
Aug 11, 2006 p
827].
- The simple rule of thumb is: melting Greenland
during 1,000 years will provide an average freshwater inflow of 0.1 Sv
(1 Sverdrup is one million cubic meters per second). Then, nobody knows
how much freshwater it takes to shut down the Atlantic circulation. A
recent model
intercomparison concluded: "The proximity of the present-day
climate to the
Stommel
bifurcation point, beyond which North Atlantic Deep Water formation
cannot be sustained, varies from less than 0.1 Sv to over 0.5 Sv."
- Over the past 30 years, Arctic surface temperatures have
increased
0.5°C per decade; September Arctic sea ice extent has decreased
7.7% per decade;
- We thus performed a sensitivity simulation with 0.1
sverdrup of water
inserted into the present-day North Atlantic over 100 years. This
freshwater forcing yields a simulated 25% slowdown of the North
Atlantic thermohaline circulation and annual cooling of 1.5-C south of
Greenland. [Otto-Bliesner]
- The present-day obliquity and precession are not in phase
as was the case for MIS-11. [ref]
As with most substances, water becomes denser with decreasing
temperature. But for fresh water this process stops at
4°C,
and as water cools further toward its freezing point, it expands to
become less dense. And unlike many substances,
frozen water is less dense than its liquid form, thus it floats.
Ocean water, with a 3.5% salinity, freezes at −1.9
°C. When
it freezes, the ice is salt free. Salt water also does not have the
density reversal of fresh water. The cold water below the ice tends to
sink, replaced by warmer water from below. The salt expelled from the
ice increases the density. also causing the water the sink. This
process brings warmer water in contact with the bottom of the ice,
reducing the rate at which the water freezes. For this reason, sea ice
does not get more than a few meters thick. [ref]
Because sea ice is floating on the ocean, the shrinking of sea ice has
little effect on sea level. Sea ice is mostly fresh water, so melting
does reduce the salinity of the ocean. Perhaps more important, the very
reflective ice surface is replaced with the strongly absorbing open
ocean, which causes further warming to occur. The following maps show
climate change in the Arctic region since 1920.
 |
(a-b)
1920-39, winter and summer, respectively. A
strong warming trend is seen in the northern Arctc mainly during the
winter season. |
(c-d)
1945-64, winter and summer, respectively. This
is a period of significant cooling. |
(e-f) 1980-1999,
winter and summer, respectively. Warming is more
global in scope during both seasons. |
Surface air temperature (SAT) trends north of 30°N
in
the
winter (NDJFMA) and summer (MJJASO) half-years for 20-year periods
representing warming, cooling and warming in the 20th century:
The following two graphs are taken from the 2004 Arctic Climate Impact
Assessment paper.
The temperature graph shows a linear rise from 1900 to
1940, a drop from 1940 to 1970, and a rise from 1970 to 2000.
The
slope of both the rise and fall is about the same. The sea
ice
graph shows no change between 1900 and mid century, then what
looks like a linear decline. The decline in summer
starts
around
1950, while the winter decline takes until 1970 to begin. But,
- Why does the temperature
rise in the early part of the century
have no effect on sea ice extent?
- Why does the decline in
summer sea ice extent begin in 1950,
while the cooling trend does not reverse itself until 1970?
- Why does the caption
attached to the graph suggest the change in
sea ice extent is accelerating? A regression taken from 1900
will
give an exponential curve. But visually at least, the decline
seems to start abruptly, and looks linear after that.



These maps show the difference between
“normal” sea ice extent
(long-term mean), and the year indicated. The long-term average minimum
extent contour (1979-2000) is in magenta. The ice extent for each year
is shown by the edge of the colored region; within that extent, color
bands show differing levels of sea ice concentration. Blue indicates
areas where concentration is more than the long-term mean; red shows
areas where concentration is less than the long-term mean. The 2005 map
shows a marked reduction in extent over the past four years, all of
which were also below average.
In 2002 and 2003, the
ice pack also experienced much lower concentrations during the minimum,
especially true north of Alaska. However, the ice cover has been much
more compact during the minimums of 2004 and 2005, yielding small
negative or even positive ice concentration anomalies within the ice
pack.
Sea Ice extent is a measure of the area that
contains at least 15 percent ice. Ice concentration is the fraction of
the actual area covered by ice compared to the total area, measured in
terms of percentage ice cover. The satellite does not pass directly
over the North Pole; this lack of data is indicated by the gray circle
in each image.
The expected time until the Arctic Ocean is ice free in summer is
decreasing rapidly:
IPCC
AR4: Sea ice is projected to shrink in both the Arctic and
Antarctic under all SRES scenarios. In some projections, arctic
late-summer sea ice disappears almost entirely by the latter part of
the 21st century.
 |
|
Spatial pattern of the percent of IPCC AR4 model
simulations (SRES A1B scenario) with at least 15% ice concentration for
March (left) and September (right),
averaged over the decade 2075 to 2084. For example, a value of 60% at a
given location means that 60% of simulations predicted sea ice. Results
are based on 11 models with realistic 20th-century September sea-ice
extent. |
Three factors make Europe’s winters milder than those of
eastern
North America at the same latitude. Winds blowing toward Europe from
the west pick up heat from the waters of the North Atlantic, which
retain far more summer heat than the interior of the North American
continent. Those balmy winds give Europe a milder, maritime climate
relative to North America’s more extreme, continental
climate.
The Gulf Stream—the tail end of a great “conveyor
belt” of currents carrying warm waters from the Southern
Hemisphere—also contributes heat to the westerly winds and
thus
to Europe. And those westerly winds tend not to blow straight out of
the west. They arrive over eastern North America from more out of the
frigid north, intensifying the continentality of eastern North
America’s climate. In contrast, Atlantic winds tend to blow
more
from the warmer climes of the south before reaching Europe.
The world’s winds carry five times more heat out of the
tropics than do ocean currents. 80% of the heat that
cross-Atlantic winds picked up was summer heat briefly stored in the
ocean rather than heat carried in by the Gulf Stream.
The ocean heat transport of the Gulf Stream was crucial to warming
Scandinavia and keeping the far northern North Atlantic free of ice. It
also warmed latitudes south of Scandinavia by 3°C on both sides
of
the Atlantic. But the wintertime temperature contrast between Europe
south of Scandinavia and eastern North America was still about
15°C. [ref]

A highly simplified cartoon of the global thermohaline circulation
(sometimes called the ‘conveyor belt’) is shown in
the
figure
above. Near-surface waters (red lines) flow towards three main
deep-water formation regions (yellow ovals) — in the northern
North Atlantic, the Ross Sea and the Weddell Sea — and
recirculate at depth (deep currents shown in blue, bottom currents in
purple; green shading indicates salinity above 36‰
[‰
means parts per mil, or in this case 3.6 percent], blue shading
indicates salinity below 34‰). A recent estimate of the rate
of
deep-water formation is 15±2 Sv
[a unit of flow expained above]
in the North Atlantic and 21±6 Sv in the Southern Ocean.
Northward heat transport into the northern Atlantic peaks at
1.3±0.1 PW (1 PW=1015
W) in the
subtropics; this heat transport warms the northern Atlantic regional
air temperatures by up to 10 °C over the ocean with
the effect
declining inland.

Return
to the Climate Change Main Page
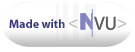