Lesson
1: The Nature of a Sound Wave
Mechanical Wave
Longitudinal Wave
Pressure Wave
Lesson 2: Sound Properties and
Their Perception
Pitch and Frequency
Intensity/Decibel Scale
The Speed of Sound
The Human Ear
Lesson 3: Behavior of Sound
Waves
Interference and Beats
The Doppler Effect and Shock
Waves
Boundary Behavior
Reflection, Refraction, and
Diffraction
Lesson 4: Resonance and
Standing Waves
Natural Frequency
Forced Vibration
Standing Wave Patterns
Fundamental Frequency and
Harmonics
Lesson 5: Musical
Instruments
Resonance
Guitar Strings
Open-End Air Columns
Closed-End Air Columns
|
Lesson 1: The Nature of a
Sound Wave
Sound is a Mechanical
Wave
Sound and music are parts of our everyday sensory
experience. Just as humans have eyes for the detection of
light and color, so we are equipped with ears for the
detection of sound. We seldom take the time to ponder the
characteristics and behaviors of sound and the mechanisms
by which sounds are produced, propagated, and detected.
The basis for an understanding of sound, music and
hearing is the physics of waves. Sound is a wave which is
created by vibrating objects and propagated through a
medium from one location to another. In this unit, we
will investigate the nature, properties and behaviors of
sound waves and apply basic wave principles towards an
understanding of music.
As discussed in the previous unit of
The Physics Classroom, a wave can be described as a disturbance that travels through a
medium, transporting
energy from one location to another location. The medium is
simply the material through which the disturbance is
moving; it can be thought of as a series of interacting
particles. The example of a slinky wave is often used to
illustrate the nature of a wave. A disturbance is
typically created within the slinky by the back and forth
movement of the first coil of the slinky. The first coil
becomes disturbed and begins to push or pull on the
second coil; this push or pull on the second coil will
displace the second coil from its equilibrium
position. As the second coil becomes displaced, it
begins to push or pull on the third coil; the push or
pull on the third coil displaces it from its equilibrium
position. As the third coil becomes displaced, it begins
to push or pull on the fourth coil. This process
continues in consecutive fashion, each individual particle acting to displace the adjacent particle;
subsequently the disturbance travels through the slinky.
As the disturbance moves from coil to coil, the energy
which was originally introduced into the first coil is
transported along the medium from one location to
another.
A sound wave is similar in nature to a
slinky wave for a variety of reasons. First, there is a
medium which carries the disturbance from one location to
another. Typically, this medium is air; though it could
be any material such as water or steel. The medium
is simply a series of interconnected and interacting
particles. Second, there is an original source of the
wave, some vibrating object capable of disturbing the
first particle of the medium. The vibrating object which
creates the disturbance could be the vocal chords of a
person, the vibrating string and sound board of a guitar
or violin, the vibrating tines of a tuning fork, or the
vibrating diaphragm of a radio speaker. Third, the sound
wave is transported from one location to another by means
of the particle interaction. If the sound wave is moving
through air, then as one air particle is displaced from
its equilibrium position, it exerts a push or pull on its
nearest neighbors, causing them to be displaced from
their equilibrium position. This particle interaction
continues throughout the entire medium, with each
particle interacting and causing a disturbance of its
nearest neighbors. Since a sound wave is a disturbance
which is transported through a medium via the mechanism
of particle interaction, a sound wave is characterized as a mechanical
wave.
The
creation and propagation of sound waves are often
demonstrated in class through the use of a tuning fork. A
tuning fork is a metal object consisting of two tines
capable of vibrating if struck by a rubber hammer or
mallet. As the tines of the tuning forks vibrate back and
forth, they begin to disturb surrounding air molecules.
These disturbances are passed on to adjacent air
molecules by the mechanism of particle interaction. The
motion of the disturbance, originating at the tines of
the tuning fork and traveling through the medium (in this
case, air) is what is referred to as a sound wave. The
generation and propagation of a sound wave is
demonstrated in the animation below.
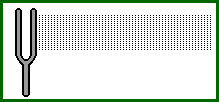
In some class demonstrations, the
tuning fork is mounted on a sound board. In such
instances, the vibrating tuning fork, being
connected to the sound board, sets the sound board
into vibrational motion. In turn, the sound board, being
connected to the air inside of it, sets the air
inside of the sound board into vibrational motion. As the
tines of the tuning fork, the structure of the sound
board, and the inside of the sound board begin vibrating
at the same frequency, a louder sound is produced. In
fact, the more particles which can be made to vibrate,
the louder or more amplified the sound. This concept was
also demonstrated by the placement of the vibrating
tuning fork against the glass panel of the overhead
projector; the vibrating tuning fork set the glass panel
into vibrational motion and resulted in an amplified
sound .
In the tuning fork demonstrations, we
know that the tuning fork is vibrating because we hear
the sound which is produced by their vibration.
Nonetheless, we do not actually visibly detect any
vibrations of the tines. This is because the tines are
vibrating at a very high frequency.
If the tuning fork which is being used corresponds to
middle C on the piano keyboard, then the tines are
vibrating at a frequency of 256 Hz - 256 vibrations per
second. We are unable to detect vibrations of such high
frequency. But perhaps you recall the demonstration in
which a high frequency strobe light was used to slow
down the vibrations. If he strobe light puts out a
flash of light at a frequency of 512 Hz (two times the
frequency of the tuning fork), then the tuning fork can
be observed to be moving in a back and forth motion. With
the room darkened, the strobe allows us to view the
position of the tines two times during their vibrational
cycle. Thus we see the tines when they are displaced far
to the left and again when they are displaced far to the
right. This is convincing proof that the tines of the
tuning fork are indeed vibrating to produce sound.
In a previous unit of The Physics
Classroom, a distinction was made between two categories
of waves: mechanical
waves and electromagnetic waves. Electromagnetic
waves are waves which have an electric and
magnetic nature and are capable of traveling through a
vacuum. Electromagnetic waves do not require a medium in
order to transport their energy. Mechanical waves are
waves which require a medium in order to transport their
energy from one location to another. Because mechanical
waves rely on particle interaction in order to transport
their energy, they cannot travel through regions of space
which are devoid of particles. That is, mechanical waves
cannot travel through a vacuum. This feature of
mechanical waves was demonstrated in class using a
segment from a laser disc. A ringing bell was placed in a
jar and air was evacuated from the jar. Once air was
removed from the jar, the sound of the ringing bell could
no longer be heard. The clapper could be seen striking
the bell. but the sound which it produced could not be
heard because there were no particles inside of the jar
to transport the disturbance through the vacuum. Sound is
a mechanical wave and cannot travel through a vacuum.
|